Introduction
The clinical findings of SARS-CoV-2 infection are heterogeneous, and the duration of viral RNA shedding varies on the age of the patient, severity of the disease, and type of the sample (1). For that reason, a comprehensive analysis of the dynamics of viral shedding is critical for describing the risk of transmission (2-5). In addition, understanding the risk of transmission is necessary to manage the cases regarding the timing of discharge or de-escalation of infection control measures (4,6).
Detection of viral RNA by reverse transcription-polymerase chain reaction (RT-PCR) is recommended for COVID-19 diagnosis and is also used for infection prevention and control precautions (7). However, viral RNA positivity does not always indicate the presence of infectious viral particles. Detection of infectious virus, also called a live virus, in specimens is considered a more informative tool for viral transmission than detection of viral RNA (8). However, few studies are showing live viruses in clinical samples (3, 9, 10). The infectious virus was detected usually in the nasopharynx, tear, and saliva of COVID-19 patients (7). Besides, limited studies reported the live virus in feces and urine (3, 11, 12). In a hamster model, the viral transmission was correlated well with the positivity of infectious virus but not with viral RNA (13).
The dynamics of the SARS CoV-2 infection differ in adults and children. Milder symptoms with prolonged viral RNA shedding may lead children to be considered a silent source of transmission (14). The key questions that are still needed to be clarified in COVID-19 adult and pediatric patients are how long a patient sheds infectious virus and the determinants of infectious virus shedding.
This study described the features of shedding of the viral RNA and live virus in samples of the nasopharynx, saliva, tear, urine, blood, and feces in both adults and children, including antibody response with their clinical and laboratory findings. We aimed to present data in a prospective cohort for the clinical practice of diagnosis and infection control measures.
Methods
Study Population
The patients diagnosed with laboratory-confirmed SARS-CoV-2 infection in Koç University Hospital between March 2020 and June 2020 were included in the study. The cases were classified as mild, moderate, severe, or critical (if they exist) according to the WHO Clinical management of COVID-19: interim guidance (15). Patients who were followed as outpatient and those discharged were followed up by phone calls until the 14th day of admission. In addition, they were invited to the hospital to give clinical samples on previously planned days. The patients with an indefinite time of illness onset were excluded.
Clinical classification, RNA testing results, demographic, clinical, laboratory, radiologic, and therapeutic information of the patients were obtained from electronic medical records. Laboratory results included routine blood biochemistry, complete blood count, lymphocyte count, interleukin-6, C-reactive protein. Chest computed tomography was obtained on admission. The dates of illness onset were also recorded at admission. While mild and some moderate cases were given hydroxychloroquine (HQ), more severe patients were given favipiravir and tocilizumab when needed, in addition to supportive therapy according to the national guide. All experiments were performed following relevant guidelines and regulations.
We collected serial samples (nasopharyngeal swabs, saliva, urine, tears, stool, and serum) from all patients every other day within the first week of their diagnosis and at the tenth, 14th, 21st days. The samples were transferred within one hour and stored at -80 ◦C.
Viral Nucleic Acid Detection
RNA from nasopharyngeal, urine, saliva, serum, tear, and semen samples were extracted manually using QIAamp Viral RNA Kit (Qiagen) with QIAcube HT (Qiagen) as instructed by the manufacturer. RNA was isolated from fecal samples with the Quick-RNA™ Fecal/Soil Microbe Microprep Kit (ZYMO Research) following the manufacturer’s instructions. Quantitative reverse transcription PCR (qRT-PCR) was conducted with primers and TaqMan probes targeting nucleocapsid N1 and RNA dependent RNA polymerase (RdRp) genes using LightCycler 480 Probes Master (Roche) in a LightCycler 480 Real-Time PCR System (Roche). Both for N1 and RNA dependent RNA polymerase (RdRp), Ct values of >40.0 were considered negative.
Viral Culture
SARS-CoV-2 RT-PCR positive specimens were cultured in the Biosafety Level-3 Laboratory (BSL-3) of Koç University İşbank Center for Infectious Diseases (KUISCID). Briefly, 2.5 x 105 Vero-CCL81 cells/ml were seeded in 96 well tissue culture plate in Dulbecco’s Modified Eagle’s Medium (DMEM) supplemented with antibiotic/antimycotic (GIBCO) and heat-inactivated fetal bovine serum (5% FBS). When the cells reach 90% confluency, 100 ml of 2-fold serially diluted stool, urine, saliva, serum, nasopharyngeal swab, and tear samples were inoculated. At seven days post-infection, 500 µl of culture supernatants were transferred to the confluent monolayers of Vero-CCL81 cells in six well plates. The cytopathic effect was examined daily up to five days; RT-PCR was performed from culture supernatants that induced visible CPE under the inverted tissue microscope (16).
Antibody Detection
In serum samples of the patients, anti-SARS-CoV-2 total immunoglobulins directed toward nucleocapsid protein of virus were measured with the electro-chemiluminescence immunoassay (ECLIA) method using Roche Elecsys Anti-SARS-CoV-2 assay via Roche COBAS 6000 analyzer (Roche, Mannheim, Germany). The CV% value for the intermediate precision in positive samples was 2.3-6.5%. The positivity was defined as a cut-off index (ratio) of ≥1.0.
We summarized and presented data using Stata V.16.0 software (Stata Corporation, College Station, Texas, USA).
Results
Eleven adult and five pediatric patients with mild, moderate, or severe COVID-19 were included. The median age was 65 years (24-91) in adults, and 64% was female. The median age was 10.2 (3-17) in children, and 60% was female. The median duration from the onset of symptoms to admission was three days.
In the adult group, three patients (27.2%) had severe, five (45.4%) had moderate, and three (27.2%) had mild disease. Eight out of 11 (73%) adults had pneumonia, and two (20%) were transferred to the intensive care unit (ICU). Two children (three months old and eight years old) had a mild infection without specific treatment. Two children (14 and 17 years old) who had a moderate infection with pneumonia were hospitalized. A nine-year-old child hospitalized for suspected juvenile idiopathic arthritis (JIA) was diagnosed with mild COVID-19 based on lack of lung involvement. Macrophage activation syndrome (MAS) was diagnosed on the day of SARS-CoV-2 positivity based on the presence of hemophagocytosis in the bone marrow. This patient was followed up in ICU.
Three children and seven (44%) adults received hydroxychloroquine. Among adults, six (55%) received favipiravir with a median duration of five days, and two received tocilizumab. On admission, 6/11 (55%) adults had lymphopenia (<1000 × 109/L). The fatality of one adult patient (91 years old) out of three (27.2%) was attributed to COVID-19. The clinical and demographic features were presented in Table 1.
In total, 408 samples from 11 adults and five children were studied. In adults, SARS-CoV-2 RNA was detected in 41.8% (23/55) of nasopharyngeal, 37.5% (21/56) of saliva, 5.6% (3/53) of feces, 5.3% (3/56) of tear, 5.5% (3/54) of serum, 3.5% (2/56) of urine samples, and none of the semen samples was positive. In children, 58.3% (7/12) of nasopharyngeal, 38.46% (5/13) of saliva, 8.33% (1/12) of feces samples were positive. However, none of the urine, tear, and serum was positive (Table 2).
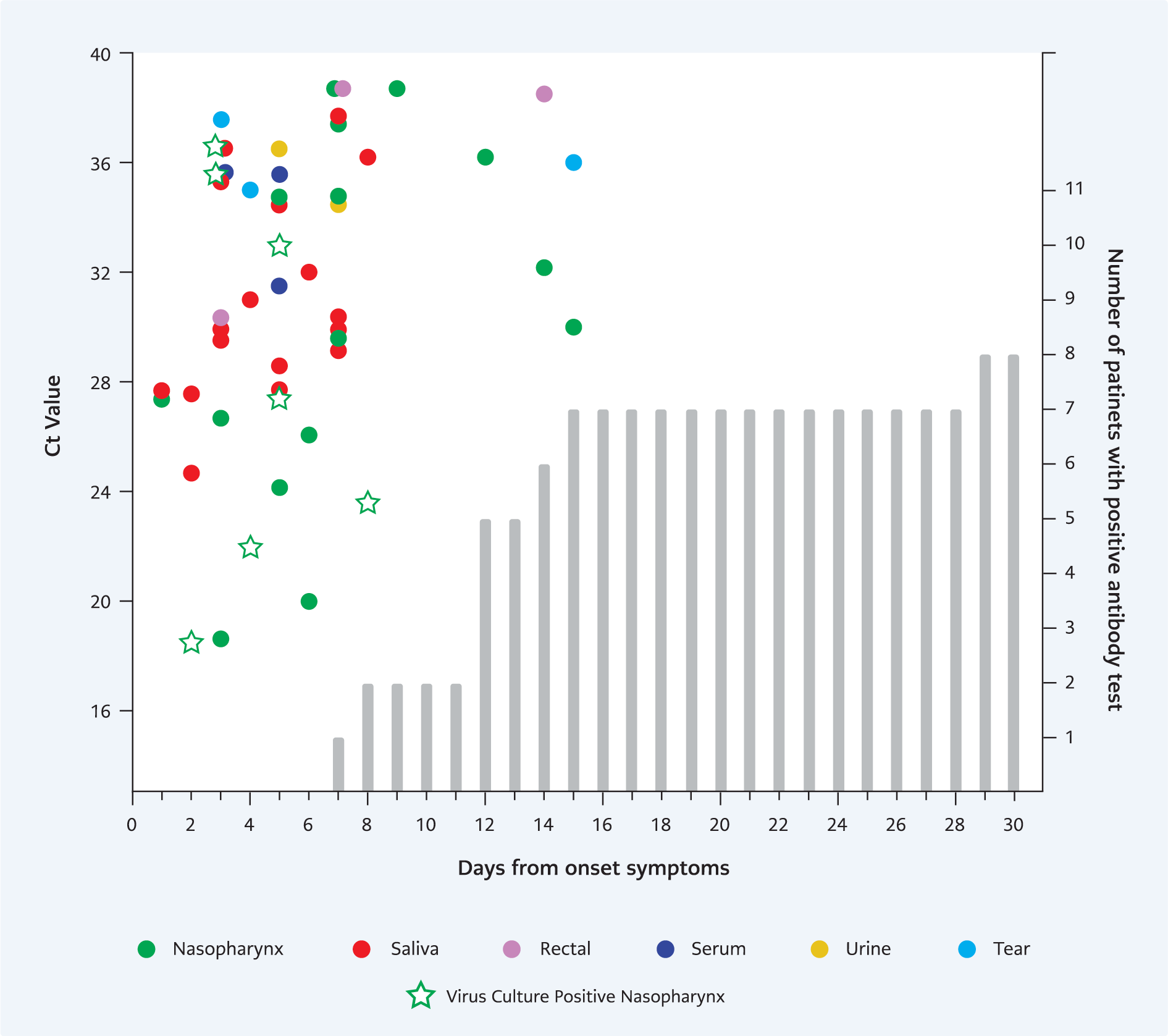
Figure 1. Viral shedding dynamics of adult patients. All PCR positive samples were cultured regardless to days or Ct values.
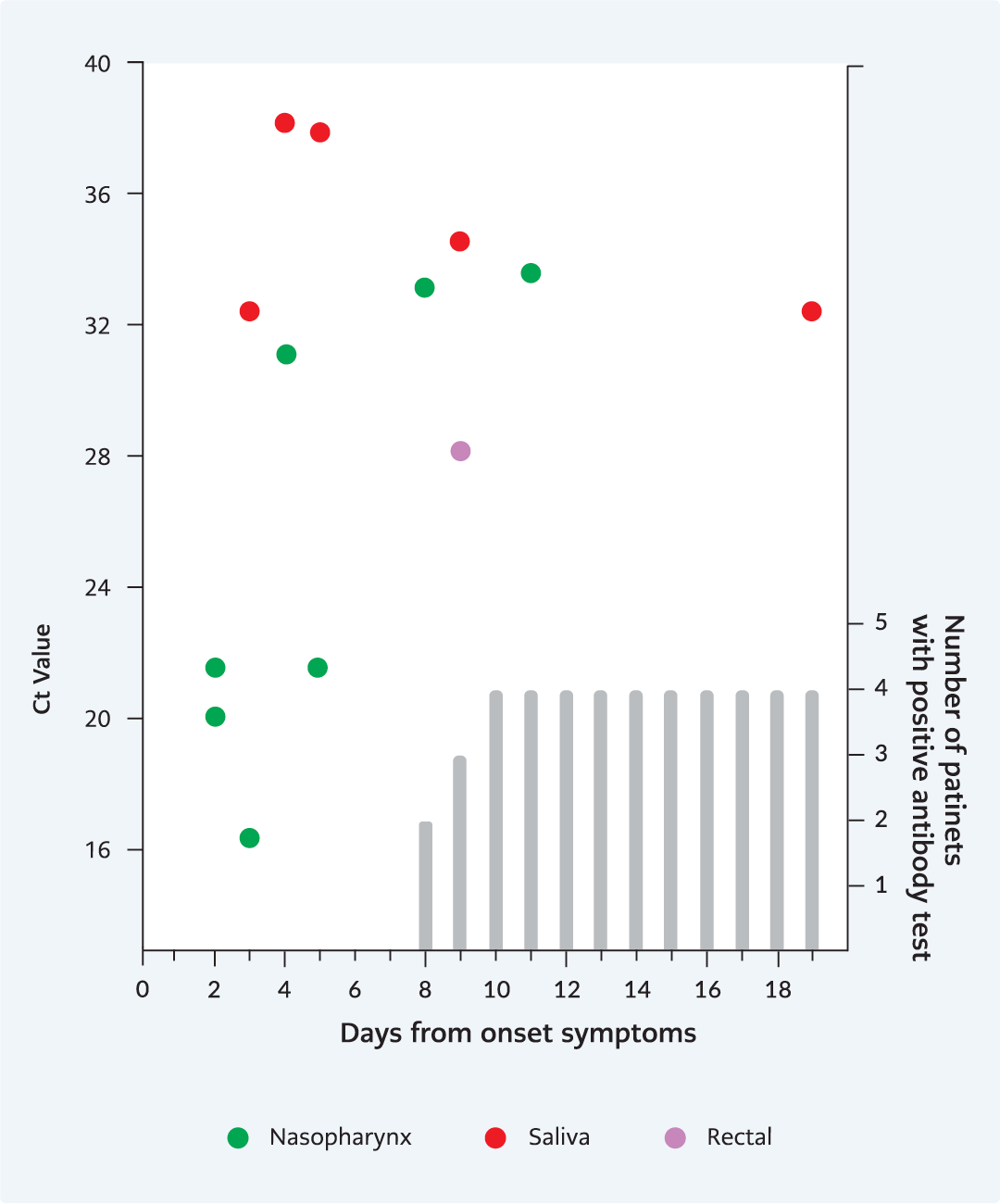
Figure 2. Viral shedding dynamics of pediatric patients. All PCR positive samples were cultured regardless to days or Ct values.
In all samples, the median duration of SARS-CoV-2 RNA shedding from the onset of first symptoms was seven (2-15) days in adults and five (3-19) days in children. Nasopharyngeal and saliva samples were positive within the five days in all patient groups. We detected the latest viral RNA in nasopharyngeal samples on day 15 in adults and on day five in children; in saliva, on day ten in adults and day 19 in children. In adults, it was positive in serum samples within five days and urine within seven days; however, we did not detect in the serum and urine of the children. Viral RNA in feces samples of adults was first detected on day three and the latest on day 14. Only one feces sample was positive on day 10 in children (Figure 1 and 2).
The viral culture was performed for all SARS-CoV-2 PCR positive specimens from adult and pediatric patients. SARS-CoV-2 was grown in seven of the 23 nasopharyngeal samples (30.4%) of adult patients. Despite their low Ct values in RT-PCR, no viral growth was detected from the sites other than nasopharyngeal ones. The median duration of infectious virus shedding in the nasopharynx was four days, and the latest virus isolation time was day eight after the symptom onset. After eight days, there was no positive culture, except one clinically severe patient despite three days of favipiravir use. All samples from children were negative.
The earliest seroconversion was detected on day seven in adults and day eight in children. The total antibody was detected in eight out of 11 adults (78%) and four out of five children (80%) within the 14 days of symptom onset. In nine (89%) adults, antibody positivity was seen on the 29th day. Two adult patients died before seroconversion on day eight and day eleven. After antibody production, the viral RNA was still detected in the nasopharynx of an adult and a child’s saliva; however, the infectious virus did not exist in these samples (Supplementary Material). No SARS-CoV-2 antibody was detected in a 74-year-old female patient’s last sample on the 16th day; she had a moderate condition with intracranial hemorrhage and diabetes. One pediatric patient with MAS and who had moderate COVID-19 did not develop an antibody response on the 29th day.
Discussion
We report infectious SARS-CoV-2 viral shedding dynamics in adult and pediatric patients in mild, moderate, and severe clinical conditions. In addition, we assessed viral shedding and infectivity in the presence of anti-SARS-CoV-2 antibody response. This information is critical for creating algorithms for diagnosis, prevention, and control precautions during the hospital stay and after discharge.
In our study, the median duration of SARS-CoV-2 RNA shedding was seven days in adults and five days in children. The longest duration of viral RNA shedding was 19 days in the saliva sample of a child. However, in other studies, the median viral RNA shedding time was reported as 17 days (17, 18). The prolonged shedding time was associated with high fever on admission, male sex, mechanical ventilation, delayed hospital admission, and hospital length of stay (17, 18). In our study, the median duration from symptom onset to admission was three days. Therefore, we may claim that short viral RNA shedding is due to patients’ earlier hospital admission, which provided effective management with supportive treatment.
In our study, the latest viral growth time for SARS-CoV-2 was eight days. In previous viral culture studies, the duration of infectious virus shedding was reported between two and eight days after onset of symptoms. Our study included mild, moderate, and severe cases. Our results were concordant with the findings of mild adult cases reported by Wölfel et al. They could not detect live viruses after eight days of the symptoms (4). Another study reported similar results without any disease severity assessment (9).
RT-PCR positivity was obtained in all nasopharynx and saliva samples within five days of infection. Ct values of nasopharyngeal and saliva RT-PCR tests were increased after the first week of the disease. In recent reports, SARS-CoV-2 RNA was detected in saliva samples with higher viral loads than nasopharyngeal samples, and saliva has a significant role in the fast-spreading nature of the epidemic (10, 19, 20). We found viral shedding among 37.5% of the adults and 38.4% of pediatric saliva samples. In one patient, the virus was detected only in the saliva sample on admission, and all other samples were negative. In our study, the latest saliva PCR positivity after symptom onset was on day ten in adults and day 19 in a pediatric patient with MAS. In another study, the viral RNA shedding in saliva was positive within 11 days (10). We did not find the infectious virus in saliva samples. Saliva viral culture positivity was reported in previous studies (21). Based on these findings, we suggest that saliva could be a good diagnostic specimen, and the risk of virus transmission by saliva may be lower than nasopharyngeal secretions.
In our patients, two adults with moderate and one child with mild manifestations had diarrhea. None of these patients’ fecal samples was found to be positive for SARS-CoV-2 with RT-PCR. The prolonged shedding of viral RNA in feces raises concerns about the timing of discontinuing precautions during the hospital stay or after the discharge. These concerns are significant for children due to immature toilet training and poorer hand hygiene. We detected viral RNA in feces of three adults on the third, seventh, and 14th days after onset of symptoms and tenth day in the pediatric patient. In a study, the viral RNA was tested positive in feces of an adult patient for 47 days (22). Children had longer fecal viral shedding than adults; in some cases shedding over 70 days was reported (23-25). However, the presence of viral RNA in feces could not be considered evidence of replicating live virus and do not confirm the potential for fecal-oral transmission. In our study, the viral culture of RT-PCR positive fecal specimens yielded no viral growth. Two studies reported the presence of the live virus in the stool samples (3, 26). However, there have been no published reports of transmission of SARS-CoV-2 through feces, but concerns about fecal transmission are still present (27). Our results suggested that the risk of fecal-oral transmission is very low, and proper hand hygiene with no extra precautions for preventing fecal transmission could be appropriate.
We found viral shedding in three out of 56 (5.3 %) tear samples in adults and one in children. We did not find the infectious virus in tear samples. An infectious virus was reported in an ocular swab specimen of a patient with conjunctivitis (12). The prevalence of shedding of the viral RNA in urine samples is very low (3, 7), and live virus in urine samples was detected in only one case report (11). We detected viral RNA in two urine samples of a patient with mild symptoms; however, live viruses did not exist in these samples.
The presence of viral RNA detection in serum of COVID-19 patients was reported; however, SARS-CoV-2 could not be cultured from serum (28). In accordance with the previous reports, we detected SARS-CoV-2 RNA 3 out of 54 (5.5 %) serum samples in two adults without any viral growth. None of the serum samples were positive for viral RNA in children.
Serological diagnosis is important, especially for patients with low viral load. The timing of antibody tests, however, is critical. In our study, the rates of total SARS-CoV-2 antibody were reached 88.8 % (8/9) in adults and 80% (4/5) in pediatric patients until the 14th day of symptom onset. Similar to our findings, Zhao et al. observed that total antibody positivity increased from the first week to 15 days of symptom onset (29). In another study conducted with 285 patients, positive antibody results were obtained at the end of the first week of the disease (30). In our study, the delayed antibody response was observed in two patients with comorbidities (diabetes and juvenile idiopathic arthritis). Some studies pointed out that delayed antibody response was associated with low viral load or impairment of adaptive immune response (31,32). In our two patients, viral RNA shedding, but not an infectious virus, lasted after seroconversion. The lack of infectious viruses in all samples after seroconversion suggested that a positive antibody test result could be used as a discharge criterion.
A key strength of our study is that we had clinical cases with molecular, and viral culture studies in the same institute. We were examining the patients in our institute, and this shortened transportation time to the laboratory. All samples were collected in transport media that do not inhibit viral growth and stored within one hour without losing infectivity. Therefore, we were able to isolate the live virus in samples with high Ct values. The number of samples per patient was 25 on average. Viral dynamics at different body sites should be further explored in larger populations of COVID-19 patients.
In conclusion, the infectivity of patients is low after eight days of symptoms. Saliva may be a good diagnostic specimen. The risk of fecal-oral transmission is very low, and strict hand hygiene measures could be preventive. Positive antibody test results could be used as a discharge criterion based on the lack of infectious virus in all samples after seroconversion.