Introduction
β-glucans area part of the fungal cell wall structure, mainly considered a potential therapeutic and diagnostic tool for invasive fungal infections (IFIs) (1). Several studies have been conducted about their effects on health (1). They have been reported to reduce blood glucose and have a strong inhibitory effect on lipid peroxidation as a regulator of blood cholesterol (2-4). In addition, these molecules’ effects in regulating the immune system and their antimicrobial, anticancer, anti-inflammatory and antiallergic properties have been proven (5, 6). Also, synergistic effects of β-glucans as antioxidant, antigenotoxic and antimutagenic activities have been reported by scientists (6, 7). In this study, the main characteristics, therapeutic options and diagnostic potentials of β-glucans were reviewed.
Methods data sources and search strategy
Databases such as PubMed, Google Scholar, Scopus, EBSCO, E-Journals, and ScienceDirect were searched using keywords such as (“Beta-glucan” OR “Fungal Beta-glucan” OR “Beta-glucan AND Diagnosis” OR “Beta-glucan AND Therapy”). The search covered literature published between December 30, 1990, and December 1, 2024. A total of 23,600 results were retrieved, including conference presentations, letters to the editor, short communications, journal articles, books, reviews, and case reports. After reviewing the titles and abstracts, 250 full-text articles were identified as relevant. Studies focusing on the effects of fungal β-glucans on the body, their anticancer properties, and their applications in diagnosis, treatment, and vaccine development were included. Ultimately, 100 articles were selected for analysis to provide evidence supporting the biological properties, immunomodulatory effects, and diagnostic and therapeutic applications of fungal β-glucans.
Chemical structure and sources of β-glucans
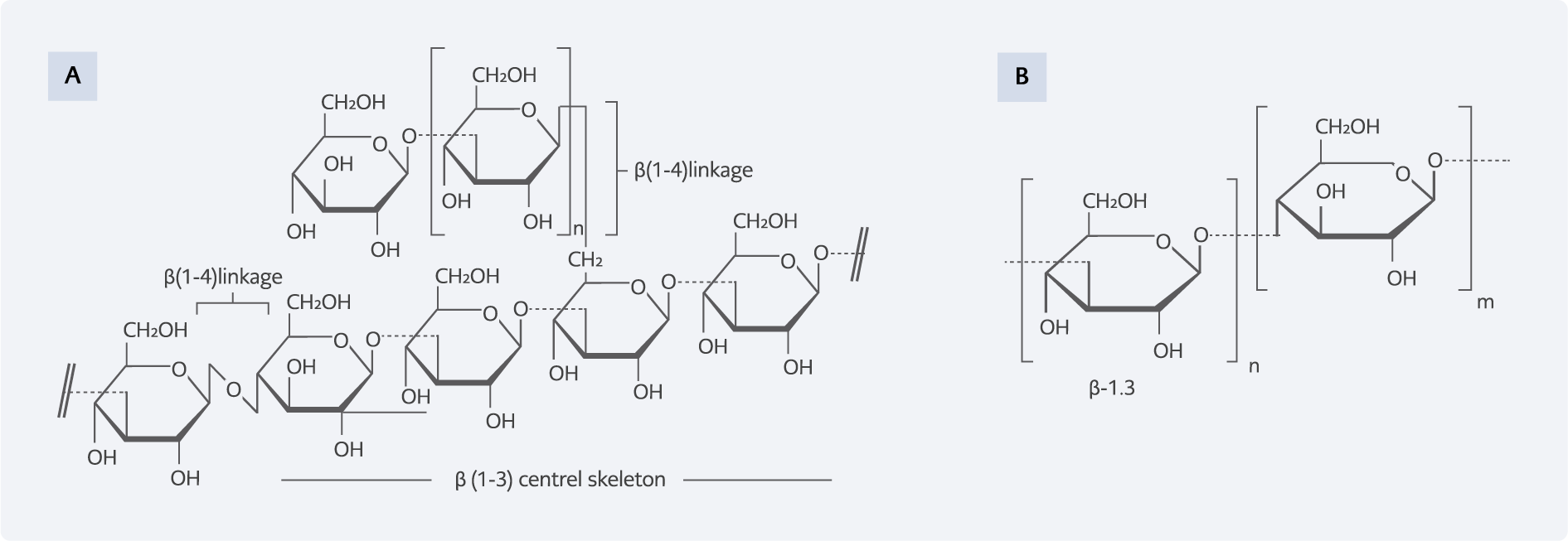
Figure 1. A. The polymer of β (1-3)-D-glycopyranosyl units with branching at β (1-6) and β (1-4)-D-glycopyranosyl units (6), B. The polymer of β (1-3)-D-glycopyranosyl units with branching at β (1-6) and β (1-4) -glycoside bonds (3).
β-Glucans are polysaccharides made of D-glucose monosaccharides linked by β-glycosidic bonds. Based on the origin of β-glucans, they differ in terms of solubility, degree of branching, mass, and molecular shape, affecting their biological activity. In general, β-glucans are divided into two isomers (1, 3: 1,4)-β-glucans based on glycosidic linkages. β-glucans in the cell wall of fungi are composed of β-D-glucopyranose molecules that are linked by β-1,3- and β-1,6-glycoside bonds and form a branched structure (Figure 1A) (6). On the other hand, β-glucans in cereals are in the form of unbranched chains consisting of β-D-glucopyranose monomers connected by β-1,3-and β-1,4-glycosidic bonds (Figure 1B) (7). These polysaccharides are present in the cell walls of some microorganisms, including fungi, bacteria, barley, wheat, some vegetables and fruits, lichens, and algae (2, 7).
The role of β-glucans in health
The health-promoting properties of β-glucans have been reported in various studies (3-5). Nowadays, it is known that zymosan obtained from the cell wall of yeasts stimulates the innate immune response due to its high concentration of β-glucan (6). It has been proven that a diet rich in β-glucans has a positive effect on human health by preventing chronic diseases such as diabetes, high blood cholesterol, obesity, cardiovascular disease, and cancer. β-glucans have effects for lowering cholesterol and blood glucose, a major factor in the prevention of obesity and metabolic disorders, as well as having antioxidant, immunomodulatory, and antitumor properties (7-9). Furthermore, β-glucans have a prebiotic effect that stimulates the growth and activity of intestinal normal flora bacteria (9, 10).
The effect of β-glucans on the innate and acquired immune system
β-glucan can modulate and stimulate the immune system (11,12). This polysaccharide leads to stimulation and activation of innate immune responses by binding to Dectin-1, complement receptor 3 (CR3), and Toll-like receptor 2 (TLR-2) on the surface of dendritic cells, neutrophils, eosinophils, monocytes, and macrophages. The binding of the Dectin-1 receptor to β-glucan leads to the activation of spleen tyrosine kinase (Syk) and nuclear factor kappa B (NF-κβ), which is followed by the production and secretion of pro-inflammatory cytokines (e.g. IL-1, IL-6, and TNF-α) and the expression of adhesion molecules (6,11). On the other hand, the binding of this polysaccharide to the CR3 receptor leads to the activation of the transcription factor NF-kβ, which is followed by the production of monocyte chemoattractant protein-1 (MCP-1) and TNF-α (Figure 2) (13). Moreover, it increases the number of lysosomes in macrophages and natural killer (NK) cells) (14). As mentioned, β-glucans can modulate innate and adaptive immunity (2). They are recognized by macrophages. These cells convert β-glucans into smaller fragments and release them outside the macrophage. β-fragments are taken up by granulocytes, monocytes, NK cells, and dendritic cells through CR3, followed by an immune response (24). Today, it is known thatβ-glucans are zwitterionic polysaccharides, meaning they carry both positive and negative charges. This unique property enables them to interact with immune cells effectively. When β-glucans are recognized by macrophages, they undergo enzymatic processing and are fragmented into smaller bioactive components. These fragments are then released from macrophages and can be taken up by various immune cells, including granulocytes, monocytes, NK cells, and dendritic cells, primarily through the CR3. In addition, β-glucans are known to activate CD4+ T cells through the major histocompatibility complex class II (MHC-II) intracellular pathway. This activation involves the binding of β-glucans to MHC-II molecules within antigen-presenting cells (APCs), facilitating the presentation of antigens to helper T cells. Furthermore, β-glucans may influence the production and metabolism of nitric oxide (NO) in immune cells, promoting an enhanced immune response. Specifically, NO, which is produced in response to β-glucan stimulation, can be converted into low-molecular-weight carbohydrates that further modulate the immune response (22, 24). Macrophages participate in wound healing through the secretion of various factors and cytokines, including TNF-α. Interestingly, β-glucan accelerates wound healing by inducing the expression of TNF-α in wound-resident macrophages (15).
B lymphocytes activated with β-glucan release IL-8, which induces neutrophil recruitment even in the absence of T cells. β-glucan stimulates B cells to produce IgG immunoglobulin (16). Many studies point to the role of β-glucans isolated from the yeast cell wall of Saccharomyces cerevisiae and Pneumocystis carinii because of its ability to stimulate immune cells (10).
Antitumor activity of β-glucans
The antitumor effect of β-glucans is associated with their ability to activate the complement system. They inhibit the proliferation of cancer cells by inhibiting tyrosine kinases, as well as inhibiting the growth of blood vessels around the tumor and inducing death through apoptosis (8, 17). β-glucans can modulate NF-κβ transcription factor activity; over activity of NF-κβ has been observed in many cancers, which causes proliferation, antiapoptotic activity, promotes angiogenesis and metastasizes (8, 18). There is a report that oral Β-glucan increased the expression of IFN-γ and the activity of NK cells, which led to a significant reduction in tumor incidence and the prevention of liver metastasis (19, 20).
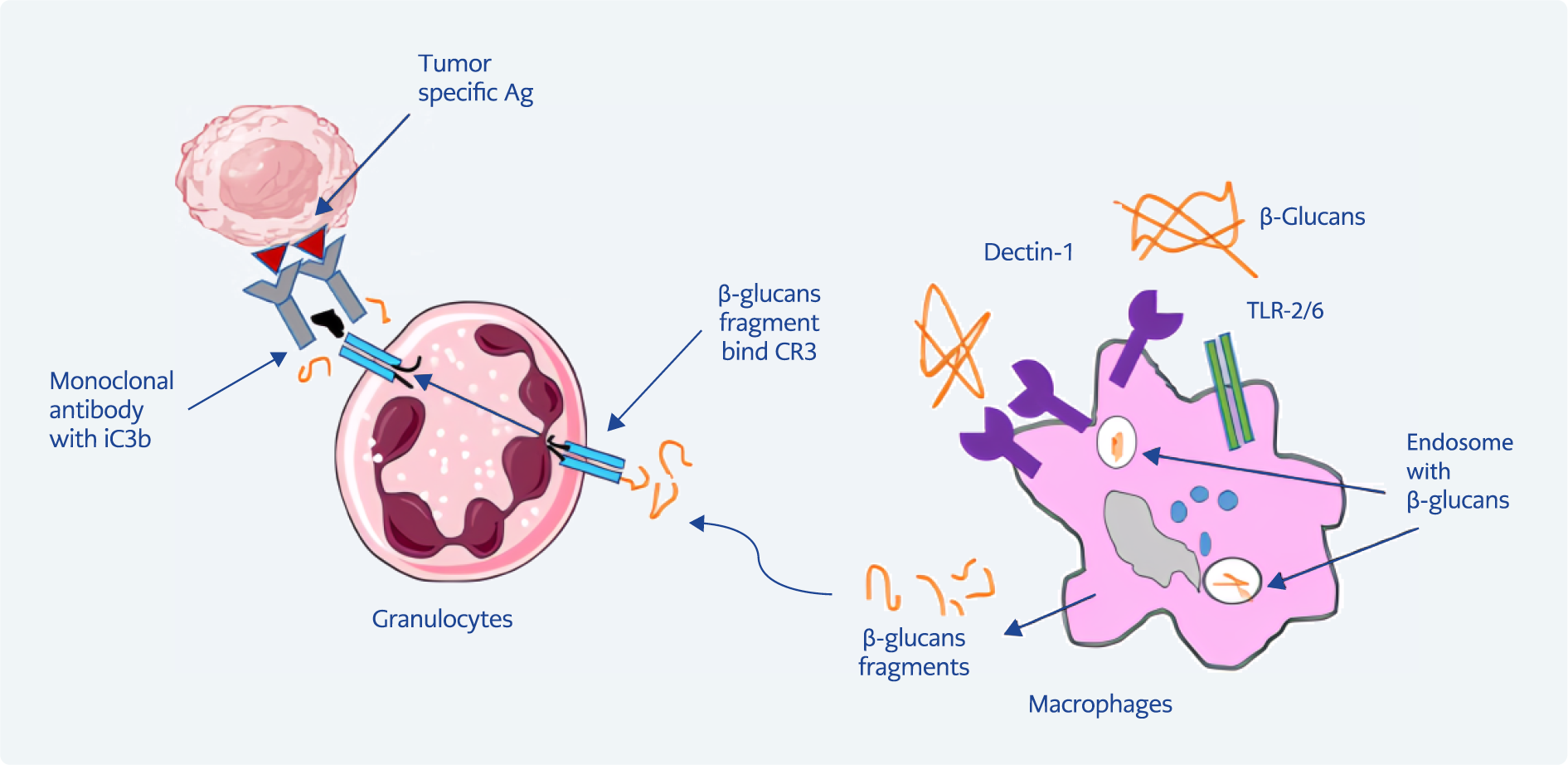
Figure 3. The role of β-glucans on tumor cells. β-glucan can bind to iC3b on surface tumor cells. Immune cells, including neutrophils and NK cells, can bind to β-glucans attached to iC3b through CR3, which leads to their activation. As a result, it leads to the lysis and destruction of tumor cells (21, 22).
Other mechanisms include active CR3 located on specific NK cells, neutrophils, monocytes, and lymphocytes. This pathway identifies opsonic β-glucans, which lead to phagocytosis and lysis cells. β-glucans bind to the CR3 lectin domain, binding to inactive 3b (iC3b) complement on the surface of cancer cells. Neutrophils containing CR3 can stimulate the lysis of iC3b-coated tumor cells (21). Similarly, most human NK cells express CR3, and it has been shown that the opsonization of iC3b-coated NK cells leads to increased target lysis (Figure 3) (22).
Improving the intestinal environment and antimicrobial effects
β-glucans can repair damage to the intestinal mucosa caused by pathogenic bacteria and maintain the integrity of the intestinal mucosal membrane by increasing neurotransmitters, including acetylcholinesterase, substance P, and serotonin, in the intestinal epithelium (23). In addition, β-glucans have various antibacterial effects, including damage to the membrane and cell wall of bacteria, penetration into the bacterial cell, disruption of cell metabolism, and increased permeability of the bacterial cell membrane (24). These polysaccharides can increase the transport capacity of the gastrointestinal tract by promoting peristalsis (25).
Various studies have been performed to prove the bacteriostatic and fungistatic effects. It has been found that β-glucans might have an inhibitory effect on the pathogenic Escherichia coli in the pig intestine (26). Furthermore, β-glucans have been effective in mice suffering from gastrointestinal infections with Candida species and methicillin-resistant Staphylococcus aureus and have increased the lifetime of mice (27).
Antioxidant properties of β-glucan
Under the influence of environmental conditions, the body’s oxidation and antioxidation systems become out of balance. β-glucans can increase the antioxidant capacity of the body by improving the activity of antioxidant enzymes such as superoxide dismutase, catalase, and glutathione peroxidase. In addition, by reducing the activity of hydroxyl radicals and superoxide anion, they increase the effect of lipid peroxidation (28). The combination of β-glucan hydroxyl groups and metal ions prevents the production of hydroxyl radicals and lipid peroxidation products (12, 28).
The effect of β-glucan on upper respiratory tract infections
Studies have shown that β-glucan consumption reduces the severity of the physical symptoms of upper respiratory tract infections (URTIs) (29, 30). The antiviral effects of β-glucan might occur through the expression of interferon-stimulated genes (ISGs) in macrophage. Therefore, it can be said that β-glucan effectively promotes the expression of ISGs by inducing IFN-γ and increasing signal transducer and activator of transcription 1(STAT1)-mediated transcriptional activity (31).
With the outbreak of COVID-19, extensive research has been conducted on various prevention and therapy options, and β-glucan has been one of the compounds of interest (32). Some studies show that β-glucan administration can help reduce the severity of the disease (32). Research and study on the exact mechanism of the immune response to SARS-CoV-2 will certainly be of great help in the treatment and prevention of this disease (33). The need for innate immune responses in COVID-19 to establish a successful immune response has been proven, and severe inflammation occurs when this response becomes problematic (33). In a study, the consumption of β-glucan supplements produced by the yeast Aureobasidium pullulans has helped to maintain the main biomarkers of IL-6, D-dimer and neutrophil-to-lymphocyte ratio (NLR) in reducing the clinical severity and mortality caused by COVID-19 compared to patients without β-glucan treatment (34, 35).
The metabolic effects of yeast β-glucans in regulating blood glucose and lipid levels add to the benefits of their use in COVID-19 because fasting blood glucose directly impacts the disease severity and mortality rate of COVID-19 (36, 37). Also, fungal β-glucans can be used as a prophylactic supplement to help fight the coagulopathy associated with COVID-19 (38).
Therefore, according to the ability of β-glucan to help recover from COVID-19, it seems that after further study in this field, it can be used as a supplement in the treatment of many respiratory and infectious diseases, especially in the treatment of COVID-19 (34).
The role of β-glucans in vaccine development
In the early 1990s, research was conducted on the effects of β-glucans and their use as adjuvants in vaccines. β-glucan with Bacillus Calmette-Guérin (BCG)-like function induces the tripartite motif family (TRIM) phenotype, which is involved in pathogen recognition and transcriptional regulation of the innate immune system. β-glucan can produce long-term TRIM against a wide range of pathogens (39, 40).
In addition, β-glucans show additive and even synergistic effects with various factors (41). For this reason, many studies have focused on the use of β-glucans in viral, bacterial, and parasitic vaccines (42-45), including hepatitis B, Toxoplasma gondii, S. aureus, and some fungal agents such as Candida species and Cryptococcus neoformans (46-51). In one experiment, whole β-glucan particles were used as part of a vaccine against systemic aspergillosis and coccidioidomycosis. The results showed that the whole β-glucan particles could be used as a basis for making a fungal vaccine (51-53). In another study, mice vaccinated with recombinant cryptococcal proteins in β-glucan particles were protected against cryptococci (54).
Oral vaccines use a variety of particle antigen delivery systems, such as immune-stimulating complexes, liposomes, microparticles, nanoparticles, virus-like particles, and β-glucans (55). Because of β-glucans size and structural similarity to natural pathogens, it increases the immune response to vaccination. On the other hand, using natural polymers to prepare antigen delivery systems in new vaccines is very important today. Meanwhile, β-glucans in the form of fine particles can not only act as immune stimulants but also as antigen carriers (Table1) (56, 57).
Since the onset of the COVID-19 pandemic, various studies have been conducted on ways to treat and prevent the disease. Considering the function of β-glucans in the immune system and their role in the production of vaccines against infectious diseases, they can be beneficial as adjuncts and improve the effectiveness of various vaccines against COVID-19. On the other hand, the vaccine effectiveness against COVID-19 decreases over time after vaccination (from 88% in the first month to 47% after five months), and it seems this decrease in immunity is because of reduced vaccine efficacy. Therefore, the use of appropriate adjuvants such as β-glucans, due to their important role in the immune system, can increase the effectiveness of vaccines. In COVID-19, since we see a severe inflammatory response and its complications in patients, the use of β-glucans is very suitable and efficient due to the regulation of the immune system and can even be used in the treatment of the disease (58). It should be noted that the existing studies related to the use of β-glucan particles in vaccines are still preliminary, and more extensive clinical trials are needed to establish the effectiveness of its use in vaccines.
β-glucans are a new tool in fungal diagnosis
Since IFI is one of the most important causes of morbidity and mortality in immunocompromised individuals and malignant patients, rapid diagnosis of infection and early initiation of antifungal therapy in these patients are of great importance (59-62). Conventional methods such as culture and histopathology are considered the gold standard for the diagnosis of IFI (63). However, these methods are invasive, time-consuming, and may be associated with false-negative results (64). Therefore, introducing new methods in the diagnosis of IFIs is of great importance. One of the methods for the early diagnosis of fungal diseases is the identification of markers of fungal infection, such as fungal nucleic acids, antigens, antibodies, or cell wall components (56, 64-67). Measurement of biological markers, such as the fungal cell wall component (1→3)-β-D-glucan (BDG), offers a non-invasive method for the diagnosis of IFIs (68, 69). BDG is present in almost all pathogenic fungal species, especially Aspergillus. Therefore, as a biomarker for the diagnosis of fungal infections, especially in certain patients at risk of IFIs, such as patients with hematological malignancies and organ transplant recipients, who are particularly at risk, measurement of β-glucan levels can help in the diagnosis and management of these infections, according to the European Society for Clinical Microbiology and Infectious Diseases (ESCMID), the European Confederation of Medical Mycology (ECMM) and the European Respiratory Society (ERS) 2017 guideline. According to the ESCMID-ECMM-ERS 2017 guideline, the sensitivity and specificity of β-glucan assays in the diagnosis of fungal infections, especially aspergillosis, strongly depend on the patient and their clinical conditions. Based on available data on different patients, including intensive care unit patients, cancer patients, patients with hematological malignancies, organ transplants, HIV, and, during the COVID pandemic, patients with COVID-19-associated pulmonary aspergillosis (CAPA), the overall sensitivity and specificity of β-glucan assays range from 77% to 99%. These results suggest that β-glucan may be useful in the diagnosis of fungal infections in these patients; however, sometimes further confirmation using other tests such as galactomannan (GM) is needed to increase the accuracy of the diagnosis (65-67, 70-74).
Also, according to the ESCMID-ECMM-ERS 2017 guideline, β-glucan is usually positive in invasive fusariosis and can be useful in the diagnosis (69). It is worth noting that in the case of candidemia, the detection of BDG in Candida spp. has been approved by the U.S. Food and Drug Administration (FDA) as an important biomarker with a sensitivity of 92% and specificity of 81% for the diagnosis of IFIs (70). The measurement of BDG, especially in HIV-infected patients, has been investigated in many studies because of the high risk of these individuals developing fungal infections, especially pneumocystis. BDG measurement has been reported to be 100% sensitive and 96.4% specific for Pneumocystis pneumonia (PCP) (75, 76). However, according to the ESCMID-ECMM-ERS guidelines, the use of BDG in the diagnosis of Mucorales and some Basidiomycetous yeasts, such as Cryptococcus spp., or Trichosporon spp., requires further investigation and marginally supports a recommendation for use (77, 78). However, the evaluation and measurement of β-glucan for the diagnosis of IFIs seems reasonable because, in various studies, the presence of BDG in the blood of patients in the early stages of the disease has been proven (61, 66, 73).
Concentrations of less than 1 pg/mL of BDG can be measured spectrophotometrically by activating factor G (horseshoe crab coagulation factor). BDG binds to the factor G subunit and activates its serine protease zymogen b subunit. The now activated factor G activates the procoagulant enzyme of the coagulation cascade (Limulus polyphemus or Tachypleus tridentatus), which in turn cleaves the chromogenic substrate Boc-Leu-Gly-Arg-P-Nitroanilide and forms a chromophore that absorbs at 450°C (Figure 4) (46, 79).
Various commercial kits, including Fungitell/Fungitell STAT/Glucatell (Cape Cod Associates, Falmouth, MA, USA), Wako/Wako-EU (Wako Pure Chemical Industries, Osaka, Japan), Fungitec G test ES/Fungitec G test MKII (Seikagaku Kogyo Co.,
Tokyo, Japan, affiliated with Cape Cod, Falmouth, MA, USA), and Dynamiker Fungus (Dynamiker Biotechnology Ltd., Tianjin, China), have been introduced in the past for the measurement of BDG in serum (80). In some studies, BDG has been investigated in urine and bronchoalveolar lavage (BAL) (79, 81, 82). One important advantage of measuring BDG in blood is that it can be detected at an early stage of infection, when the amount of fungal DNA may be very low in the early stages (75, 83, 84).
It must be considered the fact that the limitation of the BDG assay is the false-positive results. Things that can cause false positives include hemodialysis, receiving blood products such as albumin and immunoglobulins, exposure to gases contaminated with glucan and surgical sponges during surgical procedures, and patients who receive gamma globulin reagents intravenously. Nevertheless, the possibility of false positives is very low because often glucan-free equipment is available, and modern dialysis membranes manufactured from blood cells usually do not release BDG (55, 58). Therefore, by using experienced personnel and compliance with specific conditions, BDG can be a beneficial diagnostic tool in IFI, especially due to its reproducibility and short test time (79). Despite the advantages of using BDG in the diagnosis of IFIs, its measurement has not been confirmed as a routine diagnostic method for these infections (Table 2) (75, 76, 85-93). Overall, β-glucan measurement can be useful and practical as a suitable diagnostic aid due to the ease of sample collection, the short test time to determine the result, and the possibility of consecutive and repeated BDG assessments for at-risk patients.
Conclusion
Based on the study, fungal β-glucan can be considered a significant substance with numerous potential benefits for improving human health. The effects of β-glucan on immune stimulation and regulation have been confirmed in both humans and animals. In recent years, promising findings have emerged regarding the use of β-glucan in tumor treatment. Other beneficial effects include its impact on fat and sugar metabolism and its ability to lower blood cholesterol levels. Numerous studies have also been conducted on its use in diagnostics, treatment of infectious diseases, and vaccine production. In addition to its potential use in medicine, beta-glucan has wide applications in the food and chemical industries. One of the key sources of β-glucan is yeast beta-glucan. Given that yeast culture conditions are generally known, rapid and simple, the production of this substance could offer significant economic, commercial and biotechnological benefits. However, further research and clinical trials are still necessary.